Polymeric
Biomaterials For 3D Printing In Medicine
3D printing is rocking the field of tissue
engineering, regenerative medicine, and rehabilitation allowing fabrication of
modular and patient-specific scaffolds, orthoses, and prosthesis with high
structural complexity and design flexibility. Since Charles Hull initially
developed 3D printing technology in 1984 using stereolithography, 3D printing
has expanding at accelerating pace in a wide range of applications in medicine,
including craniofacial implants, dental molds, prosthetic parts, on-demand
medical equipment, surgical models, scaffolds for tissue regeneration, organ
printing, implantable biosensors, and tissue models for drug discovery.
This is due to the potential of 3D printing to
provide patientspecific designs, high structural complexity, and rapid ondemand
fabrication at a low-cost. However, one of the major bottlenecks that limits the
widespread acceptance of 3D printing for biomedical manufacturing is the lack of
polymers, biomaterials, hydrogels, and bioinks functional for 3D printing,
biocompatible, and more performing from the biomechanical point of view to meet
the different needs.
.
Although much progress has been made with 3D
printing technology, there are still remarkable issues to overcome (such as
standardization and integration of an entire biofabrication platform, software
design, capabilities of the 3D printers, reproducibility, quality by design,
biomaterials characterization, and regulatory hurdles) before it can be
recognized as a conventional biofabrication technique in medicine and reach the
medical market. Among these issues, the major bottleneck is the lack of
heterogeneity biomaterials allowing their reliable clinical use.
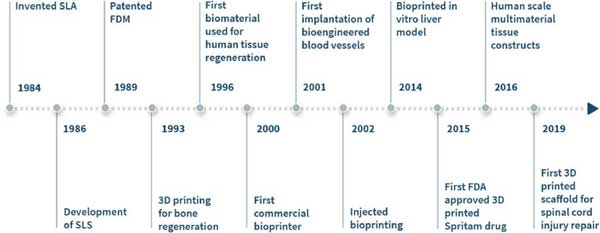
Generally, printable materials as polymers,
hydrogels, or bioinks must: (1) have adequate viscosity that allows being
printable and structurally stable, (2) have the capability to form 3D structure
within a few minutes, (3) have the possibility of being mechanically reinforced
through UV irradiation, biological (e.g. transglutaminase, sortase, tyrosinase,
lysil-amine oxidase), or chemical (e.g. Michael-type addition, thiolene
reaction, orthogonal reaction) cross-linking, (4) have tunable mechanical
properties, (5) be biocompatible, (6) have adequate degradation kinetics, (7)
form nontoxic degradation byproducts, (8) be biomimetic, and (9) be able to
control release molecules or drugs. In addition, biomaterial inks should be
easily manufactured and processed, affordable, and commercially available.
In this context, 3D printing can transform
healthcare through personalized medicine, thus improving patient compliance by
tailoring the medication to the patient. This can be achieved through on-demand
manufacturing in clinical settings to offer the best medical care .
3D printable materials
Solid polymers-based inks
Polymers are the most common types of biomaterials
used in 3D printing technologies [57, 58], since they come in the form of
filaments for fused deposition modeling (FDM), powder-beads for selective laser
sintering (SLS), solutions for stereolithography (SLA), and gels for direct ink
writing (DIW) (Fig. 2). Further, they are biocompatible, have tunable mechanical
properties, degradation rates, and can be dissolved in rapidly evaporating
organic solvents such as dichloromethane, tetrahydrofuran or dimethyl sulfoxide.
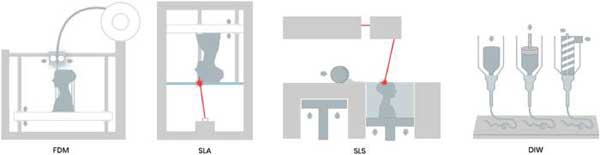
Fig. . Schematic representation of 3D printing
techniques. (a) Fused deposition modeling (FDM), (b) stereolithography (SLA),
(c) selective laser sintering (SLS), and (d) direct ink writing (DIW). Table 1.
Common polymers used in 3D printing and their properties
Name |
Melting point |
Stiffness |
Limitation |
Advantages |
ABS |
105 °C |
30 MPa |
Not
biodegradable and shrinks in contact with air. |
Good
strength and flexibility. |
PLA |
175 °C |
230 MPa |
Long-term biocompatibility. |
Good
mechanical properties; Low cost. |
PCL |
60 °C |
216 MPa |
Long
degradation time (3 years). |
Excellent rheological and viscoelastic properties upon heating; Low cost. |
PC |
110 °C |
2250
MPa |
Absorb
moisture from the air affecting performance and printing resistance. |
Tunable
mechanics and porosity. |
PEEK |
350 °C |
3.6 GPa |
High
melting point. |
High
mechanical and thermal resistance; Very strong and at the same time much
lighter than some metals. |
PP |
165 °C |
1.6 GPa |
Low
temperature resistance; Sensitivity to UV rays. |
Lightweight. |
Polyamides |
250 °C |
10 MPa |
Most
used for SLS technology. |
Good
stability, flexibility, and shock resistance. |
TPU |
235 °C |
100 MPa |
Cannot
withstand high temperatures. |
Tunable
stiffness. |
Polymeric hydrogel-based inks
Hydrogels are 3D cross-linked polymer networks,
which can absorb and retain large amounts of water (> 90%) . Commonly hydrogels
are held together by: (1) hydrogen bonds; (2) electrostatic interactions; (3)
hydrophobic interactions; (4) watermediated hydrogen bonds; (5) van der Waals
interactions; (6) covalent cross-links; or (7) a combination of the above
interactions . Hydrogels provide ideal “soft material” systems to mimic native
extracellular matrix (ECM) microenvironments due to their biocompatibility,
tunable mechanics, and degradation. Additionally, they are able to easily
incorporate bioactive cues (i.e. RGDS, IKVAV, and DGEA) or other bio-molecular
structures such as nucleic acids, fatty acids, glycans, and growth factors to
form biomimetic supramolecular scaffolds . Finally, some hydrogels are
shear-thinning and thixotropic: useful properties for bioprinting.
(Abstracted from Ref :
https://www.sciencedirect.com/science/article/pii/S2666964121000060 )
|
|